Related news by tag NANODEVICES
Science: Flatland optics with graphene
Optical circuits and devices could make signal processing and computing much faster. “However, although light is very fast it needs too much space”, explains Rainer Hillenbrand, Ikerbasque Professor at nanoGUNE and the UPV/EHU. In fact, propagating light needs at least the space of half its wavelength, which is much larger than state-of-the-art electronic building blocks in our computers. For that reason, a quest for squeezing light to propagate it through nanoscale materials arises.
The wonder material graphene, a single layer of carbon atoms with extraordinary properties, has been proposed as one solution. The wavelength of light captured by a graphene layer can be strongly shortened by a factor of 10 to 100 compared to light propagating in free space. As a consequence, this light propagating along the graphene layer – called graphene plasmon – requires much less space.
However, transforming light efficiently into graphene plasmons and manipulating them with a compact device has been a major challenge. A team of researchers from nanoGUNE, ICFO and Graphenea – members of the EU Graphene Flagship – now demonstrates that the antenna concept of radio wave technology could be a promising solution. The team shows that a nanoscale metal rod on graphene (acting as an antenna for light) can capture infrared light and transform it into graphene plasmons, analogous to a radio antenna converting radio waves into electromagnetic waves in a metal cable.
“We introduce a versatile platform technology based on resonant optical antennas for launching and controlling of propagating graphene plasmons, which represents an essential step for the development of graphene plasmonic circuits”, says team leader Rainer Hillenbrand. Pablo Alonso-González, who performed the experiments at nanoGUNE, highlights some of the advantages offered by the antenna device: “the excitation of graphene plasmons is purely optical, the device is compact and the phase and wavefronts of the graphene plasmons can be directly controlled by geometrically tailoring the antennas. This is essential to develop applications based on focusing and guiding of light”.
The research team also performed theoretical studies. Alexey Nikitin, Ikerbasque Research Fellow at nanoGUNE, performed the calculations and explains that “according to theory, the operation of our device is very efficient, and all the future technological applications will essentially depend upon fabrication limitations and quality of graphene”.
Based on Nikitin´s calculations, nanoGUNE’s Nanodevices group fabricated gold nanoantennas on graphene provided by Graphenea. The Nanooptics group then used the Neaspec near-field microscope to image how infrared graphene plasmons are launched and propagate along the graphene layer. In the images, the researchers saw that, indeed, waves on graphene propagate away from the antenna, like waves on a water surface when a stone is thrown in.
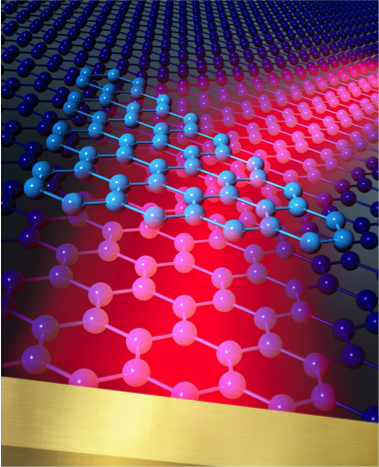
Graphic representation of the refraction of graphene plasmons – launched by a tiny gold antenna – when passing through a one-atom-thick prism
In order to test whether the two-dimensional propagation of light waves along a one-atom-thick carbon layer follow the laws of conventional optics, the researchers tried to focus and refract the waves. For the focusing experiment, they curved the antenna. The images then showed that the graphene plasmons focus away from the antenna, similar to the light beam that is concentrated with a lens or concave mirror.
The team also observed that graphene plasmons refract (bend) when they pass through a prism-shaped graphene bilayer, analogous to the bending of a light beam passing through a glass prism. “The big difference is that the graphene prism is only two atoms thick. It is the thinnest refracting optical prism ever”, says Rainer Hillenbrand. Intriguingly, the graphene plasmons are bent because the conductivity in the two-atom-thick prism is larger than in the surrounding one-atom-thick layer. In the future, such conductivity changes in graphene could be also generated by simple electronic means, allowing for highly efficient electric control of refraction, among others for steering applications.
Altogether, the experiments show that the fundamental and most important principles of conventional optics also apply for graphene plasmons, in other words, squeezed light propagating along a one-atom-thick layer of carbon atoms. Future developments based on these results could lead to extremely miniaturized optical circuits and devices that could be useful for sensing and computing, among other applications.
Original publication
P. Alonso-González1, A.Y. Nikitin1,5, F. Golmar1,2, A. Centeno3, A. Pesquera3, S. Vélez1, J. Chen1, G. Navickaite4, F. Koppens4<, A. Zurutuza3, F. Casanova 1,5, L.E. Hueso 1,5 and R. Hillenbrand 1,5. “Controlling grapheme plasmons with resonant metal antennas and spatial conductivity patterns” Science (2014), DOI: 10.1126/science.1253202
- CIC nanoGUNE, 20018 Donostia-San Sebastián, Spain.
- I.N.T.I-CONICET and ECyT-UNSAM, San Martín, Bs. As., Argentina.
- Graphenea SA, 20018 Donostia-San Sebastián, Spain.
- ICFO-Institut de Ciéncies Fotoniques, Mediterranean Technology Park, 08860 Casteldefells, Barcelona, Spain.
- IKERBASQUE, Basque Foundation for Science, 48011 Bilbao, Spain.
CIC nanoGUNE
The nanoGUNE Cooperative Research Center, located in Donostia-San Sebastian, Basque Country, is a research centre set up with the mission to conduct excellence research into nanoscience and nanotechnology with the aim of increasing the Basque Country’s business competitiveness and economic and social development.
GRAPHENEA S.A.
Graphenea is a pioneer graphene production start-up company founded in 2010 by private investors and CIC nanoGUNE. The company produces and commercializes graphene films by Chemical Vapor Deposition technology and graphene powders by Chemical Exfoliation techniques.
ICFO
ICFO is a young research institution located in Barcelona that aims to advance the very limits of knowledge in Photonics, namely the science and technology of harnessing Light. Its research programs target the global forefront of photonics, and aim to tackle important challenges faced by society at large. ICFO is focused on current and future problems in Health, Energy, Information, Safety, Security and caring for the Environment.
The researcher Estitxu Villamor got a special mention in the CAF-Elhuyar awards
Among the various categories and subcategories in the awards there is one of popularization articles based on PhD theses. In fact, Estitxu Villamor got the special mention for her article entitled "Karga-garraiorik gabeko elektronika berria" (New electronics without charge transport). The panel of judges felt that the following was worthy of mention: "The effort made by the author of the article to explain the subject in an easy-to-understand and attractive way, particularly bearing in mind that it is a highly technical subject".
Villamor defended her thesis entitled Injection, transport and manipulation of pure spin currents in metallic lateral spin valves in December 2014.
Magnetic electrodes increase solar cell efficiency
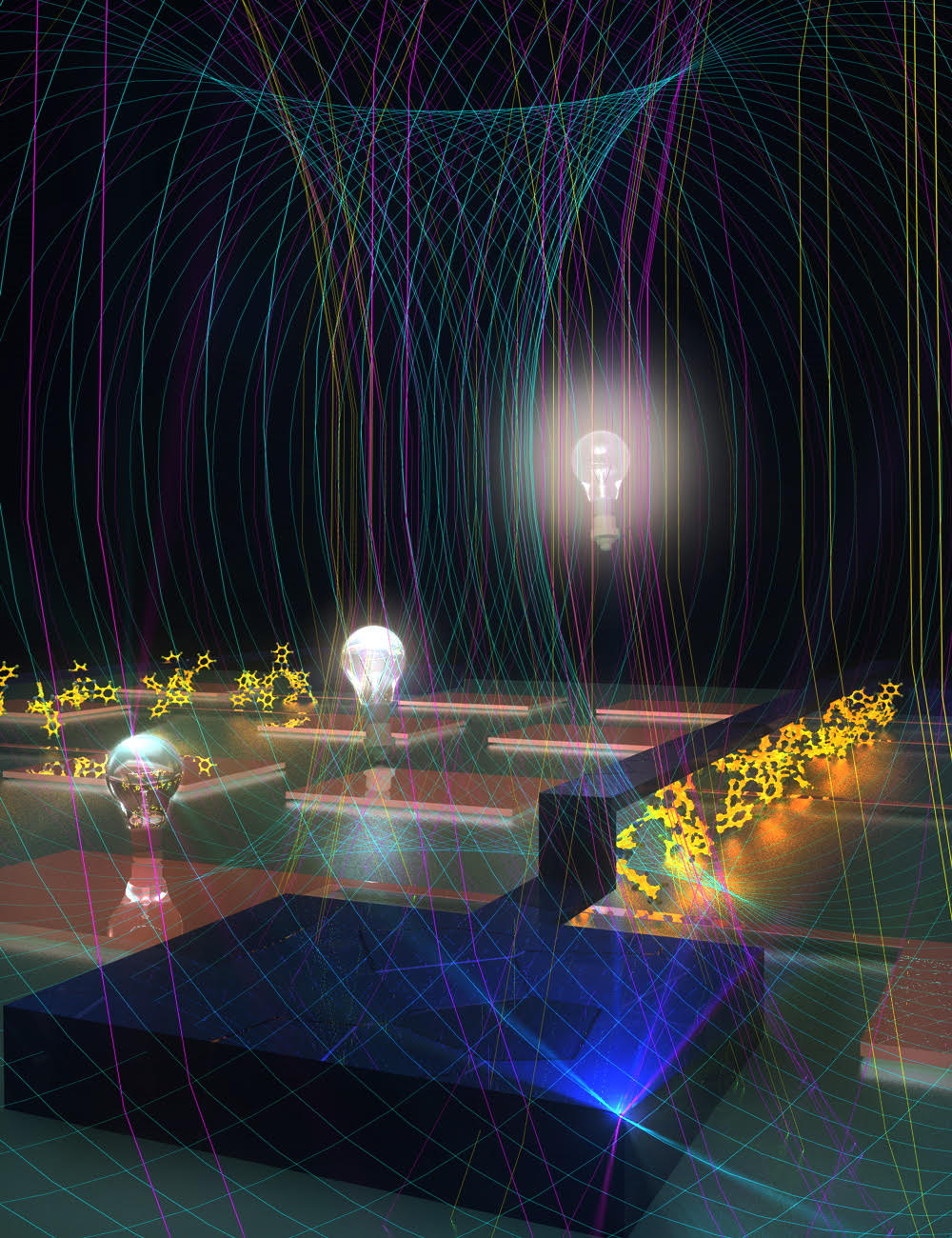
As the nanoGUNE researcher explained, “the device is simply a photovoltaic cell manufactured from an organic material —fullerene C60— and fitted with cobalt and nickel magnetic electrodes”. Fullerene C60, known as Buckyball, is a ball-shaped molecule comprising 60 carbon atoms. What is more, the magnetic electrodes produce current with an added property known as spin. The combination of both is no coincidence since fullerene is known to be a photovoltaic material that could allow the spin direction to be controlled. The use and control of this properly allows the efficiency of the solar cell to be increased, thus making it capable of generating a bigger current. As Hueso explained, “the spins of the usual solar cells are ‘disordered’ but thanks to magnetism we have managed to ‘order’ them so that a bigger current can be collected”. The researchers have confirmed that the use of electrodes of this type increases the photovoltaic efficiency of the device by 14%.
The device has another added advantage as it has been found to be capable of directly generating alternating current, which is much more useful in applications than the direct current generated by the usual solar cells, as transformers no longer need to be used. “The reversal of the current takes place in the device itself when the electrons created by the light interact with the magnetic contacts, whose spins have been ‘ordered’,” explained Hueso.
Even though it is true that the researchers have demonstrated that the use of magnetic electrodes allows the efficiency of the photovoltaic cells to be increased, they insist that they are still a long way from obtaining an optimum photovoltaic cell. With this aim in mind they are working on building similar devices using organic materials which have already shown themselves to be more efficient than fullerene. The researcher affirms that “in the future it will be possible to build a commercial device that acts as a solar module and produces alternating current directly”.
This work is the result of a piece of research funded by the Government of the Basque Autonomous Community, the Spanish Ministry for the Economy and Competitiveness, and the European Union through the European Research Council.
CIC nanoGUNE is participating in two European projects to train young researchers
Basque researchers turn light upside down
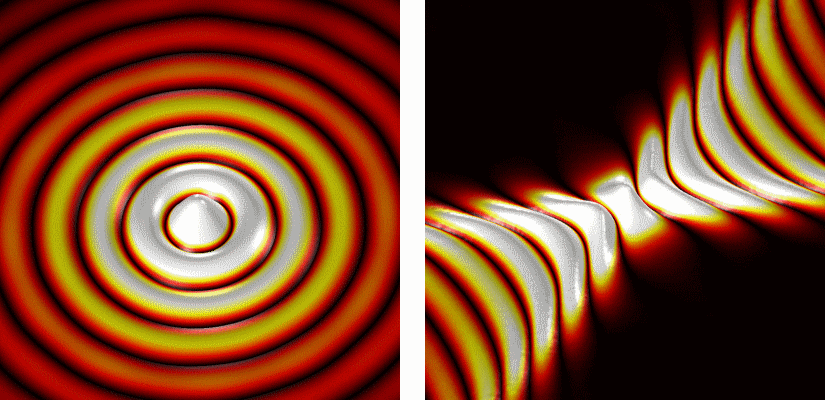
Scientists had already theoretically predicted that specifically structured surfaces can turn the wavefronts of light upside down when it propagates along them. "On such surfaces, called hyberbolic metasurfaces, the waves emitted from a point source propagate only in certain directions and with open (concave) wavefronts", explains Javier Alfaro, PhD student at nanoGUNE and co-author of the paper. These unusual waves are called hyperbolic surface polaritons. Because they propagate only in certain directions, and with wavelengths that are much smaller than that of light in free space or standard waveguides, they could help to miniaturize optical devices for sensing and signal processing.
Now, the researchers developed such a metasurface for infrared light. It is based on boron nitride, a graphene-like 2D material, and was selected because of its capability to manipulate infrared light on extremely small length scales, which could be applied for the development of miniaturized chemical sensors or for heat management in nanoscale optoelectronic devices. On the other hand, the researchers succeeded to directly observe the concave wavefronts with a special optical microscope, which have been elusive so far.
Hyperbolic metasurfaces are challenging to fabricate because an extremely precise structuring on the nanometer scale is required. Irene Dolado, PhD student at nanoGUNE, and Saül Vélez, former postdoctoral researcher at nanoGUNE (now at ETH Zürich) mastered this challenge by electron beam lithography and etching of thin flakes of high-quality boron nitride provided by Kansas State University. "After several optimization steps, we achieved the required precision and obtained grating structures with gap sizes as small as 25 nm”, Dolado says. “The same fabrication methods can also be applied to other materials, which could pave the way to realize artificial metasurface structures with custom-made optical properties”, adds Saül Vélez.
To see how the waves propagate along the metasurface, the researchers used a state-of the-art infrared nanoimaging technique that was pioneered by the nanoptics group at nanoGUNE. They first placed an infrared gold nanorod onto the metasurface. “It plays the role of a stone dropped into water”, says Peining Li. The nanorod concentrates incident infrared light into a tiny spot, which launches waves that then propagate along the metasurface. With the help of a so-called scattering-type scanning near-field microscope (s-SNOM) the researchers imaged the waves. “It was amazing to see the images. They indeed showed the concave curvature of the wavefronts that were propagating away form the gold nanorod, exactly as predicted by theory“, says Rainer Hillenbrand, Ikerbasque Professor at nanoGUNE, who led the work.
The results promise nanostructured 2D materials to become a novel platform for hyberbolic metasurface devices and circuits, and further demonstrate how near-field microscopy can be applied to unveil exotic optical phenomena in anisotropic materials and for verifying new metasurface design principles.
The research has been mainly funded by individual fellowship grants of the European Union Marie Sklodowsca-Curie Actions and the pre-doctoral research grants program of the Basque and Spanish Governments, as well as by the National Science Foundation (USA), and has been carried out in line with nanoGUNEs projects within the EU's Graphene Flagship.
NanoGUNE recruits two early-stage researchers (ESRs) for the SPEAR project
Spin Orbitronics provides a challenging and innovative framework for training early-stage researchers with excellent prospects for a career in industry and academia. In this promising area, SPEAR proposes a multidisciplinary European network, composed of 7 universities, 3 research centers and 7 small and medium sized companies, which will provide state-of-the-art training for early-stage researchers (ESRs) in the field of fundamental and applied Spin Orbitronics.
Fèlix Casanova receives the Intel Outstanding Researcher Award for the second time
Finding a replacement for today’s electronic technology to make it smaller, faster and, most importantly, more energy-efficient, i.e. so that it consumes less, is a global challenge. In recent years, Fèlix Casanova and his nanoGUNE team have been working in collaboration with the multinational Intel on a research project aimed at improving the performance and energy savings of future computers by optimizing MESO technology.
Electrical control of magnetism by electric field and current-induced torques
Albert Fert, French physicist and winner of the Nobel Prize in Physics in 2007, is one of the discoverers of giant magnetoresistance, a physical effect that revolutionised hard disk technology, allowing a huge increase in its capacity. His research enabled the capacity and applications of the hard disk drive to be increased, and he is now working towards a new generation of microprocessors t